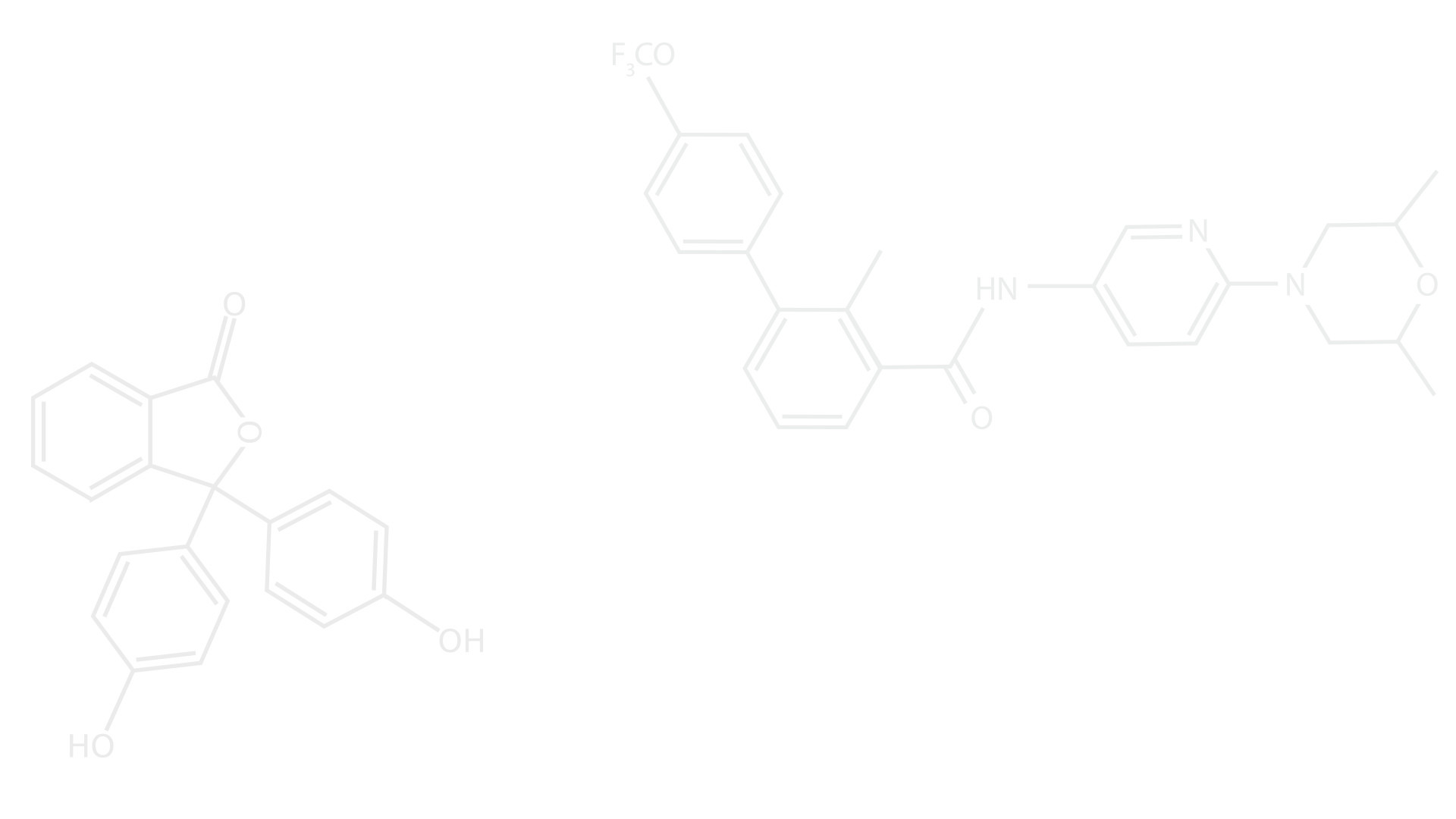
Capstone Conversation
Global warming and climate change are two major concerns facing the future of humanity and indeed all current life on Earth. Rising sea levels, mass extinctions, resource wars, and extreme weather patterns are all expected outcomes of a changing global climate. Responsible for this phenomenon are greenhouse gases (GHGs), particularly carbon dioxide, but also methane and nitrous oxide, with the recent focus being on anthropogenic (human caused) contributions of these gases and how they have disturbed the natural global balance.
​
But in order to fully appreciate the human contribution to climate change, a comprehensive understanding of the natural cycles and processes involved in GHG production and consumption is necessary. The Earth consists of a number of compartments called Earth Systems, including the hydrosphere (water bodies), lithosphere (landmasses), atmosphere, and biosphere, each one producing and consuming chemicals in various ways.
​
Focusing on one system, the hydrosphere, this is a crucial earth system in the regulation of GHG emissions. Estimates suggest approximately 2.7 Pg, that’s 2.7 trillion kilograms (10^12) of carbon are cycled through the hydrosphere each year. This is important, as carbon is an important regulator for GHG production. The situation becomes complex when one realises that the chemical, biological, and physical processes involved in the cycling of carbon, and other important chemicals (nitrogen and phosphorous being big ones) are each in turn subject to a diverse range of environmental controls. These include temperature, salinity, pH, oxygen levels, water depth, sunlight, sediment characteristics, the inhabiting organisms, wind speeds and much more.
​
The vast variability between the characteristics of individual water bodies resulting from this extensive cohort of environmental controls demands that local analysis be conducted on individual water bodies to elucidate the unique mechanisms occurring within that specific location. Doing so would not only provide a better understanding of how the controlling mechanisms work, but also help in developing much more comprehensive national emissions inventories, and therefore, allow for a more accurate assessment of the human climate impact.
​
Detailed studies into specific sites also leave us better equipped to predict how the behaviours of these specific water bodies will change and adapt as climates change. Indeed the Australian Government includes understanding the impact of change on water and marine resources as a science and research priority.
​
This then is the purpose and importance of my pilot study into the biogeochemical processes governing emissions in Smith’s Lake, an intermittently closed and open lagoon or lake (ICOLL) in lower Northern NSW. By analysing key sediment characteristics and measuring the rates and quantities at which carbon dioxide, methane, and nitrous oxide are produced, comparisons can be made from which possible relationships are inferred. GHG fluxes were measured from a seven day aerobic incubation, and compared with sediment properties to determine any linear relationships (ie. organic matter goes up, CO2 goes up). Both CO2 and CH4 had positive fluxes, expectedly correlated to increasing organic matter and total nitrogen, while N2O had a negative flux and had mostly weak correlations to measured sediment characteristics.
​
The most important finding was that Smith’s Lake had extremely low levels of denitrification (process responsible for N2O) which was exceeded by small amounts of nitrogen fixation (conversion of atmospheric N to forms useable by most organisms). This is a concern for the future health of Smith’s Lake, as denitrification is a major removal process for nitrogen, and therefore a resistance mechanism to excess nutrient levels. Ultimately however, the study serves primarily as a first stage exploration for identifying possible mechanisms and relationships existing within Smith’s Lake to then inform and focus future investigations.